Zhengtang LUO Research Group
Advanced Materials & Devices Laboratory

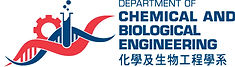
Nanomaterials
The requirement for long-range structure coherence and property uniformity for graphene-based electronics are crucial for their applications in nanoelectronics. One of our research project is to synthesize large-size single crystal graphene by Chemical Vapor Deposition (CVD) method and graphene based FET device fabrication.
In 2013, we found that trace amount of oxygen in Ar gas during the heating process helped to reduce the graphene nucleation density significantly and the following morphology characterization correlated graphene nucleation with nanoparticles larger than 20 nm. By applying this seeded growth method, we consequently achieved large single crystal graphene with grain size of sub-centimeter. We further demonstrated that we can amplify the existing graphene grain size by limiting the second seeded growth only from the graphene seed edges initiated by a mild oxidation process. In addition, we also demonstrate that similar method can be used for the preparation of large grain size bilayer graphene flakes.
Functional Polymers
In 2016, we developed a new method to engineer well-distributed dicobalt phosphide (Co2P) nanoparticles encapsulated in N, P-doped graphene (Co2P@NPG) as electrocatalysts for hydrogen evolution reaction (HER). We fabricated such nanostructure by absorption of initiator and functional monomers, including acrylamide and phytic acid on graphene oxides, followed by UV-initiated in situ polymerization, then by adsorption of cobalt ions and finally calcination to form N, P-doped graphene structures. Besides, we have also successfully fabricated other kinds of TMPs@Carbon nanostructures, including Fe2P@NPG, Ni2P@NPG and Pd5P2@NPG. More details in "Polymer-Embedded Fabrication of Co2P Nanoparticles Encapsulated in N, P-Doped Graphene for Hydrogen Generation"
Materials Chemistry
Atomic registry has strong impact on controlling the electronic structure and properties of graphene due to localized strain and localized charge distribution. However, the direct experimental evidence of the correlation between the physical structure and chemical reactivity is still lacking.
In 2015, our group reported an experimental observation that the electron transfer chemistry can significantly modify twisted bilayer graphene (tBLG) by investigating the results of chemical functionalization with diazonium salts. The relative reaction rate for diazonium salts grafting on tBLG is much higher than that on AB-stacking graphene. Gerischer−Marcus electron transfer theory analysis, along with electronic structures calculations, indicates that different reactivity mainly results from the distinct variation of Density of States distribution in the gap region. Our research suggested a venue to separate and sort different stacking modes of bilayer graphene for various promising applications in nanoelectronics.
Theoretical Modelling
We perform theoretical modeling and biophysics involving nanomaterials and biological molecules for example DNA and proteins. The present research is focused on the interaction behavior of materials and biology. These interactions could be understood and analyzed using several techniques, among them theoretical modeling and molecular simulations were used vastly and popular in scientific community.
The software's used for molecular modeling are PyMol, Chimera and VMD etc. For molecular Dynamics simulations, we use NAMD offered as open source simulation software from UIUC. The molecular simulations were performed on GPU cluster provided by HKUST. Our group had recently invested to buy new cluster services with 2XK80 Tesla Nvidia GPU, further it will be expanded. At present group research focoused on binding energy calculations between biomolecules and materials, the methods used are PMF calculations using ABF, US, MMPBSA, and REUS.
Sensors and Devices
<Not yet available>
Energy Materials
Global warming is a critical issue in recent decades due to extensive utilization of fossil fuel for energy production. To reduce the dependency on this non-renewable energy source, there is a great need to move to green economy, which will provide us sustainability in the future. In our group, we are trying to develop new high performance two dimensional (2D) materials, which could be useful for different energy applications either as catalysts or electrode materials. We specifically do research on fuel cells (hydrogen evolution, oxygen evolution), CO2 reduction, and energy storage (Li-ion battery), among others. Along with experiments, we also do theoretical calculations to predict the performance of a new material via density functional theory (DFT) by using VASP software.